Initial situation
My customer produces shock waves in a medical device through a spark gap. However, the key parameters of the medical diagnostics varied outside the tolerable limits during operation. It should be determined whether the cause lies in the ignition characteristics of the spark plug and, if so, which measures lead to improvement.
In addition, the burn-off of the electrodes was very high and limited the service life of the device. A better material should be found for the electrodes.
Realisation
For a precise analysis of the device, I proposed to my customers a simultaneous measurement of the electrical discharge and mechanical shock wave characteristics in a statically relevant number. He recorded the voltages at all electrodes and the transit times of the shock waves for four different devices, each with one hundred discharges.
In the first step, I analyzed the data using Mathematica software and calculated key parameters such as ignition delay times, maximum voltages, discharge inductance and resistance, effective discharge energy, shock wave propagation time, maximum shock wave amplitude and the maximum amplitude slope of the shock wave. The raw data was reshaped and adapted to a theoretical model developed in-house using a least square fit.
I wrote a script in Mathematica to efficiently analyze all 400 data sets. This automatically loaded all data records, extracted the key parameters mentioned above and saved the results in an Excel-readable format. I used these results to correlate the various key parameters in order to narrow down the reason for the large fluctuation in medical diagnostics.
Although the ignition delay time varied greatly, all shock wave transit time measurements remained very constant. Thus, the discharge behavior could be excluded as the reason for the high variations in medical diagnostics.
After the measurements, on my advice, the existing electrode configurations of all four devices were photographed by the customer. Large differences were found in the distances between the electrodes, which explain the sometimes long ignition delay times.
Although the long ignition delay times have no influence on medical diagnostics, they put a strain on the internal components and should be avoided. I have developed two concepts that should greatly reduce the ignition delay times with as few modifications as possible. I checked the electric fields of the concepts with a 3D simulation.
The strong burn-off of the previous electrodes is due to the manufacturing process, which I was able to find out after intensive discussions with the manufacturer. The current load integral must be known in order to select a suitable electrode material. The discharge circuit parameters (inductance, resistance, capacitance and maximum voltage) were determined from the measured voltages using an FIT over time. With these parameters and an LT-Spice model, the discharge current and the current load integral could be calculated. This allowed the expected service life of the electrodes to be specified for various materials.
By selecting the optimum material and optimizing the shape of the electrode tip, it was possible to increase the service life of the electrode by a factor of 1.8. In addition, a reproducible shape of the electrode tip can be achieved with this material and a processing step can be saved.
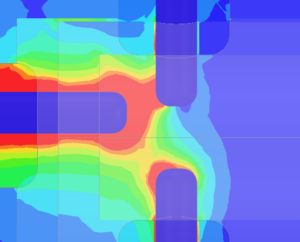
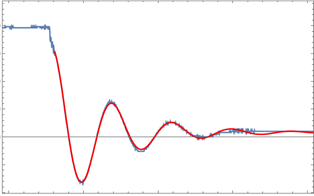
Targets and key figures
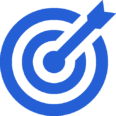
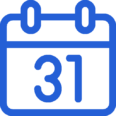
April – June 2024
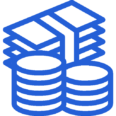
Budget 16’000 CHF
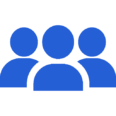
Independent project work
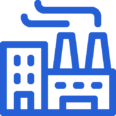
Medical technology